Scientific Studies
Thank you for visiting Copan’s Scientific Studies Library, your online resource for proof sources related to Copan products as well as many other aspects of sample collection and transport, automation, food and pharmaceutical testing, forensic and genetic collection and more!
Some of the studies in this database are independent scientific studies performed by third parties, while some of the studies were partially or entirely funded by Copan. In addition to peer reviewed research this library also contains posters, which were presented at various scientific meetings, and may not have been subject to peer review.
Some of the studies found here may contain information about off label product use. Studies, product claims and availability may vary from country to country, and they are subject to varying regulatory requirements. Always refer to product labeling, package inserts and safety data sheets for the appropriate use of the products.
Recent Scientific Studies
Study Validates Effectiveness of Copan FecalSwab® for Enteric Pathogen Detection on the BD MAX™ System
August 26, 2024
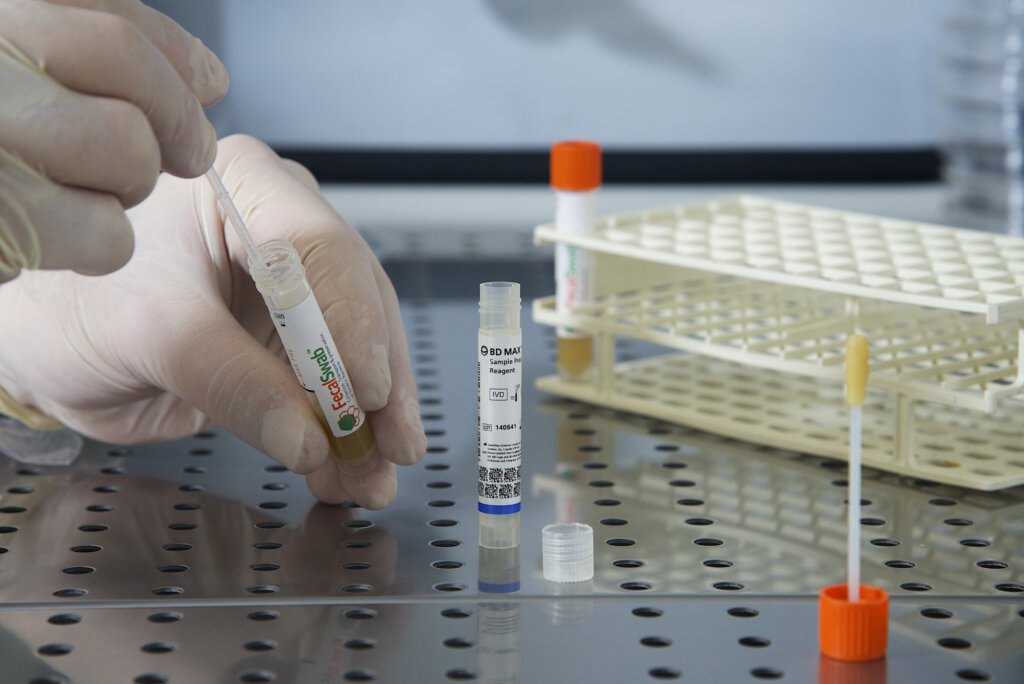
Study Presented at CACMID 2024 Highlights Copan’s UniVerse® as a Game-Changer in Clinical Molecular Specimen Preparation
July 22, 2024
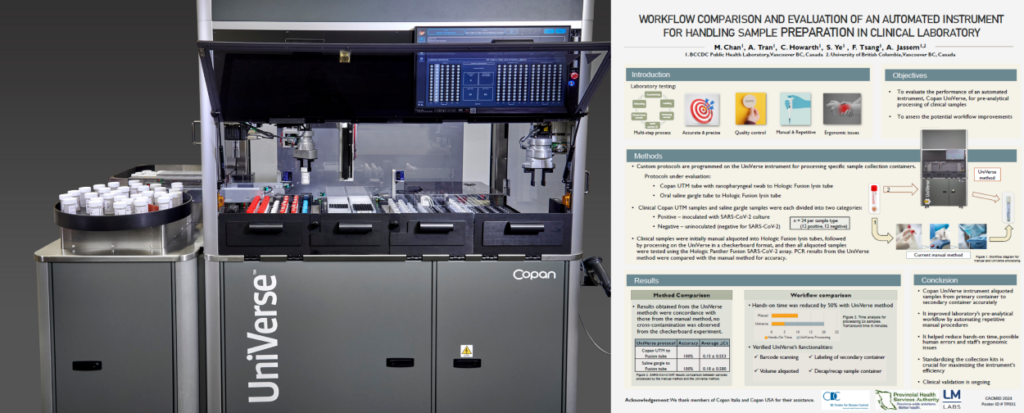